Ultraviolet Radiation and Aquarium
Lighting
By Dana Riddle
Riddle Aquatic Laboratories
Ultraviolet (UV) radiation has been a source of controversy among reef
hobbyists. The effects of natural solar radiation have been
well studied and are a matter of record. However, few studies of UV produced
by artificial lighting are available to aquarists. (Our initial studies
of lamps commonly used in reef aquaria applications and their ultraviolet
radiation output were published in Aquarium Frontiers in 1996 and were
meant to complement the previous work of ( Bingman, 1995.)
This article will present the results of our on-going testing in this important
aspect of reefkeeping and supplements works by Bingman as well as Joshi
and Morgan (1998).
Before continuing, perhaps a definition of ultraviolet radiation is
in order. Ultraviolet energy is classified into three categories;
UV-A is radiation just below the violet portion of the visible spectrum
and consists of those wavelengths between 320 and 400 nanometers (nm).
UV-A is invisible to the human eye and is the least destructive.
UV-B wavelengths (280 – 320 nm) are biologically destructive and, through
overexposure, cause erythrema (sunburn).
The third UV category consists of wavelengths between 200 and 280 nm
and is referred to as UV-C. The Earth’s atmosphere absorbs
UV-C produced by the sun and therefore is not found naturally.
However, UV-C can be produced by artificial means. Arc welding produces
UV-C; so do certain specialty electrical lamps (such as those employed
by hobbyists for the sterilization of aquarium water).
Our discussion will focus on UV-A and UV-B. (We should note
again that UV radiation is not visible to the human eye. It is not,
as many hobbyists believe, related to the “blueness” of the light.)
Fluorescence is the phenomena in which UV light is absorbed by certain
pigments and emitted in the visible portion of the spectrum.) We
measured the output of popular aquarium lamps using an instrument called
a radiometer. Manufactured by UVP, Inc. (Upland, CA) this radiometer uses
separate probes – one to measure UV-A (calibration point of 365 nm); the
other senses UV-B (calibration point of 310 nm). This instrument reports
UV energy in units of microwatts per square centimeter per second - µW·cm²·sec,
or, for brevity, µW. The readings from this instrument
are NIST-traceable. For comparisons, this instrument reports maximum temperate
latitude UV-A as about 2,000-2,100 µW; UV-B is about 1,000 µW.
Measurements taken in Hawai’i (Makena Landing, Maui) at noon on a sunny
November day showed UV-A as 1,260 µW and UV-B as 960 µW.
Why should aquarists concern themselves with ultraviolet radiation?
Don’t most metal halide lamps use a glass envelope to “shield” the UV-producing
inner arc tube, thereby reducing UV to “safe” levels? And,
after all, fluorescent lamps don’t produce any UV, correct? Additionally,
isn’t UV the reason that corals are colorful at all? We investigated
these issues and report our findings here, much of it for the first time.
Ultraviolet Output of VHO Fluorescent Lamps
It isn’t uncommon to see lamp advertisements touting that fluorescent
lamps don’t make any ultraviolet energy. Not so. The amount generated may
be low, but it is there. This UV energy is produced by an electrical arc
between the electrodes located at either end of the lamp tube. Phosphors
within the arc tube absorb the invisible UV and fluoresce –“emit” - most
of it in the visible spectrum. However, some UV is transmitted through
the glass tube.
Figure One shows the UV-A distribution of 4-110 watt URI lamps (two
“Actinic-Day”; two “Super Actinic”). These lamps were suspended
4.5” above the UV sensors. The internal reflective surface was painted
white and there was no lens or “splash guard”. As one can see, the amount
of UV energy generated by these lamps is relatively low.
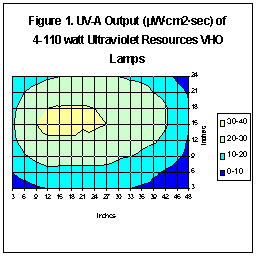
Ultraviolet Output of Compact Fluorescent Lamps
Compact Fluorescent lamps are relatively new to the aquarium trade.
Advertisements routinely claim that these lamps produce more “light” than
comparable VHO lamps, (which our research confirms). They also produce
more ultraviolet radiation. Figure Two shows the UV-A energy distribution
pattern of 4-55 watt lamps marketed by Hamilton Technologies.
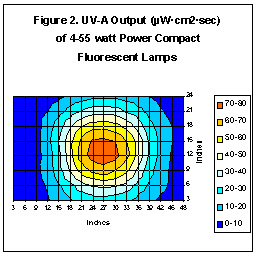
Figure Three shows the UV-A energy distribution pattern of 4-96
watt lamps distributed by CustomSeaLife.
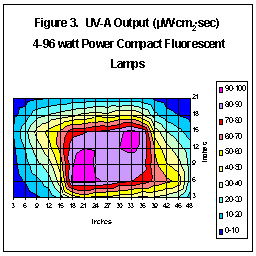
We should warn against making direct comparisons of Figures 1-3. These
measurements were not made under standardized conditions (in these cases,
reflector material and lamp height above sensor). We wish to
demonstrate only that fluorescent lamps generate UV, albeit in relatively
small amounts.
Ultraviolet Output of Metal Halide Lamps
Metal halide lamps have many myths surrounding them. They are variously
noted to produce “high” amounts of UV energy, or “none” due to absorption
by protective glass envelopes. We address this issue and also demonstrate
that seemingly small variables can make profound differences in the amount
of UV energy actually reaching a coral within an aquarium (generally, these
variables also make a difference in the amount of photosynthetically active
radiation – PAR – making it into an aquarium).
Evaluation of Shielding by Metal Halide Glass Envelopes
It is a common belief among hobbyists that the outer borosilicate glass
“globe” absorbs most or all UV energy generated by metal halide lamps (and
others). This seems reasonable since most lamps used in the hobby carry
an “R” warning (see 'Markings' below). We wondered if the glass used in
American, Japanese or German metal halide lamps possessed different UV
transmission qualities. To this end, we developed a simple experiment
to test metal halide glass envelopes. A mercury vapor “sun lamp” was used
as the UV source. We then placed shards of glass from shattered outer
envelopes of Coralife and Radium lamps over our UV-A and UV-B sensors.
An unbroken Iwasaki lamp was also tested and, obviously, since this envelope
was intact, these readings represent the UV energy traveling through two
layers of glass. Table One reports the somewhat surprising results
of our testing.
Table One
Percentage of UV Energy Transmitted by Lamp Glass
Lamp |
UV-A (%) |
UV-B (%) |
Coralife |
85.3 |
60.3 |
Iwasaki |
79.2 |
61.9 |
Radium |
83.7 |
62.9 |
As we can see, the glass envelopes of these lamps transmit most UV energy.
So, the next logical question asks us to investigate the amount of UV energy
produced by commonly used metal halide lamps.
Evaluating Metal Halide Lamps
In order to make meaningful comparisons of different lamps; we must
standardize testing parameters. For our tests, we obtained new lamps
and “burned” them continuously for 100 hours using the same ballast. We
burned the lamps continuously for 8 hours after changing orientation (vertical
to horizontal or vice versa). We tested all lamps using the same ballast
of appropriate wattage, lamp (arc tube) height above the sensor was 9.5
inches, no reflectors or shields were used, all testing was conducted in
a darkened room, etc.
We found metal halide lamps to produce varying degrees of UV radiation.
However, these measurements should not be considered absolute. Quality
control, at its best, allows a wide degree of variance from lamp to lamp,
even in the same production run. We checked three new (100 hour burn-in
period), “identical” Aqualine Buschke 175 watt 10,000K metal halide lamps
for maximum UV-A and UV-B output. Testing was conducted under standardized
conditions (height, lamp orientation, etc). Table Two reports this
information.
Table Two
UV Output of Three “Identical” Metal Halide Lamps
Lamp |
UV-A (µW) |
UV-B (µW) |
#1 |
1,259 |
48 |
#2 |
749 |
Not Tested |
#3 |
1,539 |
83 |
Does UV distribution vary from lamp to lamp? To determine this, we tested
four new (100 hr. burn-in) Coralife 400 watt 10,000K lamps for UV distribution.
We measured UV radiation across a grid system (24”X48”) for a total of
128 readings each. Distribution patterns were similar between the four
lamps and Figure Four is typical of these patterns. (UV output was also
fairly consistent, with a difference of 7.8% between the lamps producing
the most and least radiation. Compare this to the single maximum readings
in Table Two).
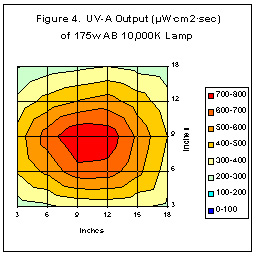
Influences of Lamp Burn Position
Generally, fluorescent lamps are mounted horizontally (the electrodes
are on a horizontal plane) and the operation position (called the burn
position) is not much of an issue. The same is true for power
compacts (although it is possible to mount these lamps vertically).
With metal halides, burn position is critical for proper lamp performance
(PAR output, color temperature) and lamp life. Metal halide lamps can be
mounted vertically, horizontally or at an angle.
Markings Found on Metal Halide Lamps
Most metal halide lamps have markings somewhere on the glass envelope.
It is important to know how to read these. They provide information for
proper operation and lamp life. Disregard markings on the metal
base; these generally denote quality control information, such as date
of manufacture.
Example: Coralife 10000K MH175/U/ED18 USA R
Coralife – Maker or Distributor
10000K – Kelvin rating (K): 10000, a very blue spectrum, higher numbers
(14000, 20000, etc.) indicate that the light produced by the lamp is “bluer”
or “cooler”. A lesser number (5000, 6000, etc.) indicated that
the light is more yellow or “warmer”. Sunlight is generally
rated at 6500K.
MH - Metal halide
175 - Lamp Wattage
U - Universal burn position.
ED - Lamp Shape (Elliptical Dimpled) and Maximum Diameter (in
this case, lamp diameter is 2.25 inches).
18 - 18-8ths, which equals 2.25.
USA – Country of Origin.
R - Indicates lamp will not extinguish if the outer envelope is broken.
Interestingly, these lamps carry a warning about potential UV exposure
should the outer envelope break, even though this envelope does little
to attenuate UV when it’s intact! A “T” indicates that the
lamp will extinguish if the outer envelope fails.
Some lamps will not have all the above information; others will have
different codes. Here are some examples:
Common Markings Designating Lamp as a Metal Halide:
MH, MT, “Halogen-Metalldampfl”.
Common Kelvin Ratings:
4,500, 5,500, 6,000, 6,500, 10,000, 12,000K, 14,000, 20,000 K.
DX = Daylight Deluxe (about 6,000 - 6,500K)
DL= Daylight (about 6,000 – 6,500K)
BDX = Blue Deluxe
Lamp Shapes:
T = Tubular.
T7=23 mm diameter;
T8= 25 mm diameter.
T lamps have no outer envelope; they are a naked arc tube.
BD17/BD54 = Elliptical Dimpled, 2.125 inch diameter.
E17N/E54N = Narrow Neck, 2.125 inch diameter.
E28 or ED28/E90= Elliptical Dimpled, 3.5 inch diameter.
ED18= Elliptical Dimpled, 2.25 inch diameter.
ED37/E120 = Elliptical Dimpled, 4.625 inch diameter
BT28= Blown Tubular, 3.5 inch diameter
BT37/BT120= Blown Tubular, 4.625 inch diameter
BT56/BT17= Blown Tubular, 7 inch diameter (usually 1,000 watt lamps)
Common Wattages:
50, 75, 100, 150, 175, 250, 400, 1000
Burn Positions (failure to burn a lamp in the proper position can reduce
lamp life and affect “light” and UV output, or the lamp may not start at
all):
U: Universal, lamp base vertical (up, down), horizontal, angled – (e.g.,
no specific burn position necessary).
BU: Base Up
VBD, VBU, BU/BD or BUD: Base Up or Down (within 15° of vertical)
BD: Base Down
V: Vertical
HBU: Horizontal to Base Up
HBD: Horizontal to Base Down
HOR or BH: Horizontal
Influences of Luminaire Shapes and Reflective Surfaces
Reflector materials commonly include painted surfaces, cast aluminum,
polished aluminum and mirrored surfaces. Each of these reflects
UV radiation differently. Table Three lists the relative reflective qualities
of three materials.
Table Three
Reflective Qualities of Various Materials*
Material |
UV-A |
UV-B |
White** |
Poor |
Poor |
Mirror |
Good |
Good |
Polished |
Good |
Best*** |
*Results based on percentage increase of UV.
**Polished Aluminum Surface painted with Flat White Enamel.
***”Best” does not necessarily mean “good!”
The shape of the reflective surface can also profoundly affect the amount
UV radiation falling upon a given point. Generally, pendant
(vertical) mounts focus PAR and UV more effectively than horizontal mounts.
Some pendants are much more effective than others are. Figures Five
and Six illustrate how differently shaped (and constructed) pendants can
focus UV radiation. These tests used the same lamp (Coralife
400w 10K), ballast (CWA), height (9.5”), etc.; the only difference was
the luminaire.
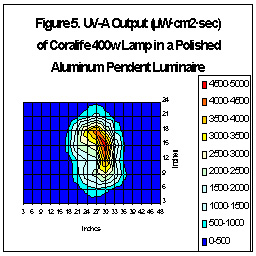 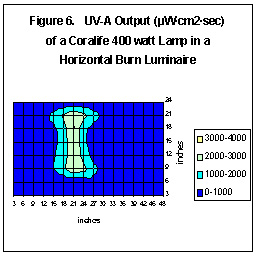
As we can see, the luminaire can make a dramatic difference - maximum
UV-A output is almost 5,000 µW – 2.5 times the amount the amount
of maximum temperate latitude UV. (If this luminaire were lowered to 4.5”
above the water, there would be a smaller area of higher UV, something
around 14,000 µW - 7X the amount of temperate sunlight.)
We are basing this projection on data we gathered using jigs and our UV
sensors.
Table 4
µE·m2·s
|
Height = Inches
|
50 |
33 |
75 |
28.125 |
100 |
24 |
200 |
16.4375 |
300 |
13.375 |
400 |
11.625 |
500 |
10.375 |
600 |
9.4375 |
700 |
8.75 |
800 |
8.1875 |
900 |
7.6875 |
1000 |
7.1875 |
1100 |
6.875 |
1200 |
6.5625 |
1300 |
6.4375 |
1400 |
6.25 |
1500 |
6.0 |
1600 |
5.75 |
1700 |
5.625 |
1800 |
5.5 |
1900 |
5.375 |
2000 |
5.25 |
2500 |
4.625 |
Lamp Warm Up and UV Production
We were curious about the production of UV energy during a metal halide’s
warm up stage (called lamp strike). We reasoned that invisible
UV energy would be produced during the strike and gradually subside as
the halides within the arc tube vaporized and began to emit visible light.
Our test results showed the maximum UV-A produced during lamp strike to
be 17% higher than a fully warmed lamp; UV-B was 100% higher.
Figure Seven shows the results.
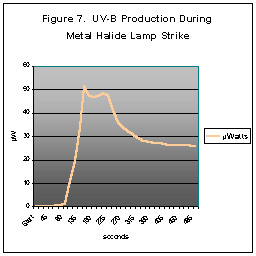
Focusing of UV Radiation by Waves
Any visitor to a pool or shallow body of water has seen chain link-like
patterns of light dance across the bottom. These lines are
called the “caustic network” (hobbyists often, and incorrectly call these
“glitter lines”. Glitter is a reflection of light such as one
sees on the water surface at sunrise or sunset). The caustic network is
caused by waves focusing and defocusing of light (these waves act
like concave and convex lenses). Lynch and Livingstone (1995) give a simple
formula to determine the depth at which light is at its focal point – Multiply
the wavelength (crest-to-crest or trough-to-trough) of the water wave by
5. Example: If an aquarium has surface waves with a wavelength of about
0.5 inches (which is typically for many aquaria), then we could expect
the focal point to be about 2.5 inches below the surface. We checked the
focusing of UV in an aquarium and found measurements of up to 5% higher
at the predicted focal point than above the water’s surface. The same held
true for PAR. We believe our instruments’ reports of peak readings are
very conservative. Stramski and Legendre (1992) report the flashes are
of only 5-20 milliseconds in duration and their sensor detected light pulses
five times greater than surface readings.
Discussion
Ultraviolet radiation was generated by all light sources that we investigated.
The actual amount produced depended upon many factors, including lamp type,
lamp orientation and even small manufacturing differences between lamps.
When we consider the significant differences that the reflector shape and
construction add to the mix, it is apparent that only generalities can
be made as to what an aquarist can expect from his or her particular light
system. In some applications, we contend that there be reason
for concern, especially when we consider that UV-A and UV-B can be significantly
higher during lamp strike and then focused by waves on the water surface.
Biological Effects of Ultraviolet Radiation
It has long been recognized that UV radiation penetrates natural waters
(Jerlov, 1950; 1976). Lewis (1995) noted that reef organisms employ
several mechanisms to protect themselves from UV: avoidance, protection
and repair. Obviously, many corals colonize areas with relatively
high UV energy, so they have measures to protect themselves. Shibata
(1969) found UV-absorbing pigments in certain Staghorn corals (Acropora)
and Cauliflower corals (Pocillopora) as well as a blue-green alga.
He speculated that a compound he called S-320 (meaning it absorbed UV energy
at and around 320 nm) might protect marine organisms from ultraviolet energy
penetrating shallow water. An even earlier researcher, (Kawaguti, 1944)
speculated that some coral pigments offered protection against “strong
sunlight.” Jokiel (1980) was perhaps the first to report UV
as an important ecological factor on shallow water tropical ecosystems.
Together, these papers along with others suggested that UV radiation could
have deleterious effects on coral reef communities.
Perhaps the best single source of information on this subject was published
in 1995. Entitled “Ultraviolet Radiation and Coral Reefs;”
this work contains many papers on the effects of UV on corals, zooxanthellae,
plankton, etc. Clearly, these present a convincing argument that ultraviolet
radiation can have a major impact on coral reefs in many different ways.
What effects can UV have on coral reef organisms? A review
of the literature suggests that the effects can be numerous and far-reaching.
Ultraviolet radiation can play a role in determining the survival of
corals from their very beginnings. Baker (1995) reported that
UV-A inhibited larvae settlements of the Cauliflower coral (Pocillopora
damicornis); Gulko (1995) demonstrated that UV-B damaged the gametes of
the Plate coral (Fungia scutaria) - possibly explaining why corals often
spawn in darkness.
UV (or UV in synergy with high PAR, temperature, etc.) is known to inhibit
photosynthesis. Glynn et al found a combination of high
temperature (31°C) and high UV (A & B, 25-30% of direct exposure)
caused a high mortality rate among Acropora vallida specimens (significantly
more Pocillopora specimens survived this treatment). Lewis (1995)
suggests that the zooxanthellae in the stony coral Montipora verrucosa
may be damaged by exposure to ultraviolet radiation. Shick (1991) reported
that the octocoral Clavularia (commonly called Star Polyps by hobbyists)
exhibited a 50% decrease in photosynthesis when exposed to “high” levels
of UV-A and UV-B. Hohlbauch (1995) reported the same effect
on the Plate coral (Fungia scutaria). Gleason and Wellington (1992) used
an underwater spectroradiometer to determine that increased dosages of
UV could induce bleaching (loss of zooxanthellae) in the stony coral Montastrea
annularis. UV was found to reduce the amounts of photosynthetic pigments
and cause photoinhibiton (a reduction in the rate of photosynthesis) in
the red alga Porphyra leucosticta (Figueroa et al, 1997). Donkor
and Hader (1997) found that UV-B radiation caused bleaching of photopigments
in the cyanobacterium Phormidium. The list of references could go on and
on. It should be sufficient to say that ultraviolet radiation
is potentially harmful to natural and captive ecosystems.
“Filtering” of Ultraviolet Radiation by Aquarium Water
Many aquarists believe that yellowing substances normally found in
aquarium water will quickly absorb UV. We tested for UV transmission in
aquarium water of several “colors” and report the results of two tests
here. We used submersible UV probes, a sliding depth gauge,
a Hach Color test kit and a “sunlamp” for the UV source. Aquarium water
was “colored” with skimmate from a foam fractionator (protein skimmer)
filtered through Whatman #4 paper. Figures 8 and 9 illustrate
the water transmission properties.
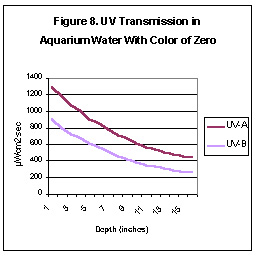 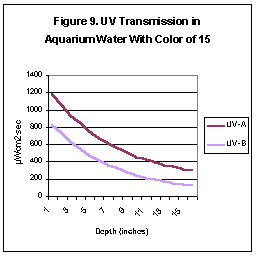
As can be seen, UV energy falls off rather rapidly. However,
under certain conditions (high wattage, reflector material with high UV
reflectance, etc.), it is possible for UV-A radiation levels at the bottom
of an aquarium to exceed UV found at the ocean’s surface.
Corals’ Natural Sunscreens
Many shallow-water organisms contain natural sunscreens to protect
them from UV radiation. These include holothurians (sea cucumbers,
Shick et al, 1992), algae (Shibata, 1969), corals (Shibata, 1969; Dunlap
and Chalker, 1986; WuWon et al, 1995, 1997) and others (Dunlap, Chalker
and Bandaranayake, 1988). These sunscreens are amino acids, more specifically,
mycosporine amino acids (MAA’s). There are many MAA’s; each of these absorbs
different UV wavelengths. For example, an MAA called S-320 absorbs UV at
and about 320 nm; others have absorption maxima of 310 nm through 360 nm.
These substances are colorless and block only UV – they allow visible radiation
(PAR) to pass.
Factors Affecting MAA Concentrations in Coral Tissues
Increased UV levels (due to the thinning of the earth’s ozone layer)
have lent an urgency to the study of UV-absorbing compounds.
Kuffner et al (1995) analyzed the MAA content of several coral genera (Pocillopora,
Porites and Montipora spp.). They found that MAA concentrations
were inversely proportional to depth with its associated lower dosage of
UV energy. (We must note that other researchers have suggested that water
motion and other environmental factors may trigger the production of MAA’s.)
Gleason (1993) transplanted brown Porites specimens from areas of relatively
low UV energy to areas of higher UV and found these corals to exhibit reduced
growth and possible photoinhibiton (he felt that UV energy in the range
of 310-350 nm was responsible). He also found that corals could
proportionally alter the amounts of certain MAA’s in response to UV levels.
MAA’s may be obtained through the diet (as in the case of the non-hermatypic
coral Tubastrea); it is also possible that zooxanthellae translocate MAA’s
to the coral animal. In any case, the coral’s response to increased
UV may be an increase in MAA’s within its tissues. This response
is not instantaneous. (The analogy of a pasty-white sunbather
trying to get a golden tan in one day is appropriate!)
In nature, corals exist in a relatively stable UV environment.
If we were to graph diurnal UV doses, we would see a bell curve, that is,
UV increases as the sun climbs toward its apogee and then decreases towards
sunset. UV may peak during a given month (depending upon latitude)
but these changes are incremental and predictable. Such is
not the case in aquaria.
UV Depreciation of Aquarium Lamps
During the course of our investigations, we’ve checked the time-course
UV output of many lamps. Figures 10 and 11 show this UV-A output
of two metal halide lamps. As can be seen, one lamp’s UV output
dropped over a period of time. Interestingly, UV output increased
rather dramatically in the other lamp. Our point is that neither
of these lamps offers a stable UV environment. Increases will
occur either during normal operation of the lamp or when the hobbyist “suddenly”
replaces the lamp. (We’ve found fluorescent lamps lose their UV output
over time as well.)
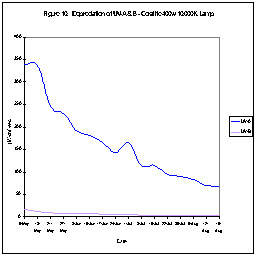 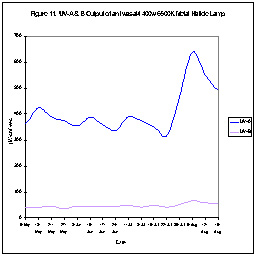
This leads us to an important question – Is there a risk of eye damage
from an aquarium using a light source with high UV output?
We do not claim to be ophthalmologists and are not qualified to give medical
advice. We can report the results of our testing.
Using a glass aquarium, we found UV-A levels of up to 70% being transmitted
through the glass above the water surface. Under certain conditions,
we believe this level of radiation could exceed that of natural sunlight.
Most acrylics attenuate UV quite well; however, comprehensive testing is
required before a definitive statement can be made.
Coral Coloration and UV Radiation
It is quite popular to believe that increased coral coloration is a
response, at least in part, to UV radiation. Our experiences
indicate that some corals will turn green as a response to increased UV.
However, we have observed many corals (especially Acroporids, Pocilloporids,
etc) exhibiting vivid coloration when maintained for years under conditions
of practically no UV (~1 µW UV-A; <1 µW UV-B).
Figures 12 and 13 show two Acropora specimens maintained under such low
UV levels (however, visible light – PAR – levels were quite high).
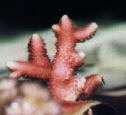 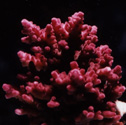
Review
These short articles have shown that all the lamps we tested produced
UV radiation. Metal halide lamps’ outer glass envelopes only
weaken UV; they do not eliminate it. The actual amount of UV
produced by a metal halide lamp depends upon many factors such as lamp
wattage, lamp orientation and arc tube construction (universal burn position
versus bottom up or bottom down). Profound differences can
exist between “identical” lamps. More importantly, the shape
(along with the type of reflective surface) can focus UV energy into “hot
spots” where the UV energy exceeds that found in nature. Even
higher UV energy is produced during lamp strike and water surface waves
can further focus this radiation. Seemingly small differences
(such as lamp height) can also have a major impact upon the amount of UV
entering an aquarium. With so many variables involved, it is
difficult, if not impossible, to know how much UV is being produced without
actually making measurements.
We have seen that UV radiation has the potential to damage corals and
other coral reef inhabitants. Many reef animals can produce
natural sunscreens (MAA’s) to protect themselves against UV but MAA concentrations
are possibly a response to the amount of UV to which they are subjected.
This is an important point since UV production among all tested lamps was
not consistent and can change dramatically during normal operation or when
the lamp is changed.
Our experiences suggest that coral coloration is a response to PAR levels,
not UV. In short, we find no reason to subject reef aquaria
to high UV levels.
Shielding Aquarium Lamps
Hobbyists may think their aquaria are doing fine under unshielded lamps.
We suggest that they can do even better by removing UV radiation.
We’ve checked many “plastics” and Table Five lists the transmission properties.
Table Five
Transmission Properties (Percentage) of Various Materials
Material
|
Thickness (Aprx.)
|
PAR (%)
|
UV-A (%)
|
UB-B (%)
|
Lexan |
1/8" |
86 |
<1 |
<1 |
Lexan Solar |
3/32" |
89 |
<1 |
<1 |
"Green" Glass |
3/16" |
91 |
73 |
3 |
AtoHaus UF-3** |
3/16" |
90 |
5 |
<1 |
Unknown Plastic*** |
3/16" |
92 |
85 |
35 |
*Window glass purchased from a hardware store.
**We’ve found UF-3 to retain its UV-screening properties even after
a decade of use. Coralife and Hamilton reportedly use this
material in their luminaires.
***From a Giesemann aquarium luminaire. Material was yellowed
and may have lost its UV screening properties as a result of aging.
We strongly recommend that an appropriate material be used to attenuate
(weaken) UV energy produced by all aquarium lamps and especially metal
halide and other lamps (such as mercury vapor). Since UV acting in
synergy with temperature damages some corals, removing UV may allow an
aquarium to run at slightly higher temperatures (thus lessening the use
of expensive water chilling). There are tradeoffs. Some photosynthetically
active radiation (PAR) will be lost as a result and the shielding material
will require periodic cleaning. Based on our observations
(and through discussions with literally hundreds of hobbyists across the
country), we believe that these tradeoffs are well worth the benefits.
We wish to thank Omer Dersom, John Lipsey and Ron Feigen for supplying
some of the lamps and/or equipment used in our testing.
References
Baker, A., 1995. The effect of UV on the settlement of
the planula larvae of Pocillopora damicornis. In: Ultraviolet
Radiation and Coral Reefs. D. Gulko and P.L. Jokiel (eds.), HIMB
Technical Report #41.
Bingman, C., 1995. The effect of activated carbon treatment
on the transmission of visible and UV light through aquarium water. I:
Time-course of activated carbon treatment and biological effects.
Aquarium Frontiers 2(3): 4,5,16-19.
Donkor, V. and D. Hader, 1997. Ultraviolet radiation effects
on pigmentation in the cyanobacterium Phormidium unicinatum.
Acta Protozoologica 36:49-55.
Dunlap, W.C. and B.E. Chalker, 1986. Identification and
quantification of near-UV absorbing compounds (S-320) in a hermatypic scleractinian.
Coral Reefs, 5:155-159.
Dunlap, W.C., B.E. Chalker and W.M. Bandaranayake, 1988.
Ultraviolet light absorbing agents derived from tropical marine organisms
of the Great Barrier Reef, Australia. Proc. 6th Int. Coral
Reef Symp., Australia. 3:89-93.
Figueroa, F.L., S. Salles, J. Aguilera, C. Jiménez, J. Mercado,
B. Vinegla, A. Flores-Moya and M. Altamirano, 1997. Effects
of solar radiation on photoinhibition and pigmentation in the red alga
Porphyra leucosticta. Marine Ecology Progress Series, 151:81-90.
Gleason, D.F., 1993. Different effects of ultraviolet radiation
on green and brown morphs of the Caribbean coral Porites astreoides.
Limnol. Oceanogr., 38(7): 1452-1463.
Gleason, D.F. and G.M. Wellington, 1993. The intensities
of ultraviolet radiation that induce bleaching of a Caribbean coral.
Proc. 7th Int. Coral Reef Symp., Guam. 1: 71. (Abstract).
Gulko, D., 1995. Effects of ultraviolet radiation on fertilization
in the Hawaiian coral Fungia scutaria. In: Ultraviolet Radiation and Coral
Reefs. D. Gulko and P.L. Jokiel, eds. HIMB Tech.
Report #41.
Hohlbauch, S.V., 1995. The metabolic response of Fungia
scutaria to elevated temperatures under various UV light regimes. In: Ultraviolet
Radiation and Coral Reefs. D. Gulko and P.L. Jokiel, eds.
HIMB Tech. Report #41.
IES Lighting Handbook, 1984. John Kaufman and Jack Christensen,
Editors. Illuminating Engineering Society of America, New York.
Jerlov, N., 1950. Ultraviolet radiation in the sea.
Nature (London) 166: 111-112.
Jerlov, N., 1976. Marine Optics. Elsevier Oceanography
Series, Elsevier Sci. Publ. Co., New York. 231 pp.
Jokiel, P.L., 1980. Solar ultraviolet radiation and coral
reef epifauna. Science, 207:1069-1071.
Joshi, S. and D. Morgan, 1998. Spectral analysis of metal halide
lamps used in the reef aquarium hobby. Part 1: New 400-watt lamps.
Aquarium Frontiers On-line. November. 8 pp.
Kawaguti, S., 1944. On the physiology of reef corals VI.
Study on the pigments. Palao Trop. Biol. Sta. Study, 2:617-674.
Kuffner, I.B., M.E. Ondrusek and M.P. Lesser, 1995. Distribution
of mycosporine-like amino acids in the tissues of Hawaiian scleractinia:
a depth profile. In: Ultraviolet Radiation and Coral Reefs.
D. Gulko and P.L. Jokiel, eds. HIMB Tech. Report #41.
Lewis, S., 1995. Response of a stony coral to short-term
exposure to ultraviolet and visible light. In: Ultraviolet
Radiation and Coral Reefs. D. Gulko and P.L. Jokiel, eds.
HIMB Tech. Report #41.
Lynch, D. and W. Livingstone, 1995. Color and Light in Nature.
Press Syndicate of the University of Cambridge. New York. 254
pp.
Shibata, K., 1969. Pigments and a UV-absorbing substance
in corals and a blue-green alga living in the Great Barrier Reef.
Plant and Cell Physiol., 10:325-335.
Shick, J.M., W.C. Dunlap, B.E. Chalker, A.T. Banaszak and T.K. Rosenzweig,
1992. Survey of ultraviolet radiation-absorbing mycosporine-like
amino acids in organs of coral reef holothuroids. Mar. Ecol.
Prog. Ser., 90:139-148.
Shick, J.M., Lesser, M.P. and W.R. Stockaj, 1991. Ultraviolet
radiation and photooxidative stress in zooxanthellate anthozoans: the sea
anemone semoni and the octocoral Clavularia sp. Symbiosis 10:
145-173.
Stramski, D. and L. Legendre, 1992. Laboratory simulation
of light-focusing by water surface waves. Mar. Biol., 114:341-348.
Wu Won, J., B. Chalker and J. Rideout, 1997. Two new UV-absorbing
compounds from Stylophora pistillata: sulfate esters of mycosporine-like
amino acids. Tetrahedron Letters, 38(14):2525-2526.
Wu Won, J., J. Rideout and B. Chalker, 1995. Isolation and
structure of a novel mycosporine-like amino acid from the reef-building
corals Pocillopora damicornis and Stylophora pistillata. Tetrahedron
Letters, 36(29):5255-5256.
This article is the 'paid' property of Aquarium Design and absolutely
no commercial reproduction is permitted without written permission in advance.
Direct your comments or questions to the author at
RiddleLabs@aol.com
|